A keen-eyed observer can spot about 4,500 stars in the sky; our galaxy, the Milky Way, is estimated to contain more than 300 billion of them. It’s obvious to any stargazer on a clear night that we live in a universe vast beyond imagining, glittering with worlds besides our own—and yet, as far as we know, Earth is the only planet to have developed living creatures of any kind. Intelligent life is an unrepeated experiment, with a sample size of one. Could we really be alone?
Sadly, we cannot yet venture out into the galaxy in pursuit of new life and new civilizations, Star Trek-style. What we can do, confined to our rock spinning through space, is speculate using the data available to us. We already know many things about the overall size and shape of the universe; with rigorous calculation, and a touch of guesswork, we can begin to put some numbers on the question of alien life.
The Drake equation is designed to do exactly this. A simple probabilistic formula, with seven parameters, it nevertheless holds profound implications for astronomy, and—given the correct inputs—could tell us how many alien civilizations are out there right now.
The search for extraterrestrial intelligence
Humans have been trying to find extraterrestrial life ever since we invented the most rudimentary tools to do it. 19th-century astronomers spent long hours peering at Mars through their telescopes, hungry for any sign that it might be home to a civilization like ours; this led to fanciful tales of artificial “canals” crisscrossing the planet’s surface.
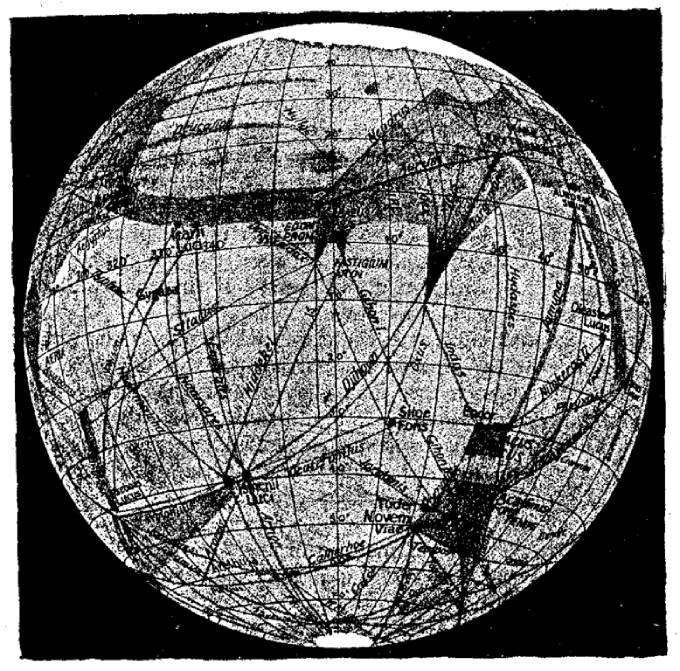
After the invention of radio, astronomers had a whole new technology with which to step up the search for extraterrestrial intelligence (SETI). In 1924, US Navy stations attempted to pick up radio signals during a close encounter with the planet Mars, and in 1960, an experiment called Project Ozma used a West Virginia radio telescope to listen in on Epsilon Eridani and Tau Ceti, two nearby stars suspected of harboring life. Spearheading this latter project was a young researcher named Frank Drake—a central figure in today’s story.
Frank Drake
Frank Drank (1930-2022) was born to a middle-class family in Chicago, Illinois. At the age of eight, he was already wondering about life on other planets—if life on Earth was the result of random chance, he thought, then what was stopping it from arising elsewhere in the universe? Thoughts of alien intelligence followed him through college and beyond; at 30, while he was working for the National Radio Astronomy Observatory at Green Bank, West Virginia, he persuaded his colleagues to try and listen in on extraterrestrial signals. This was Project Ozma.
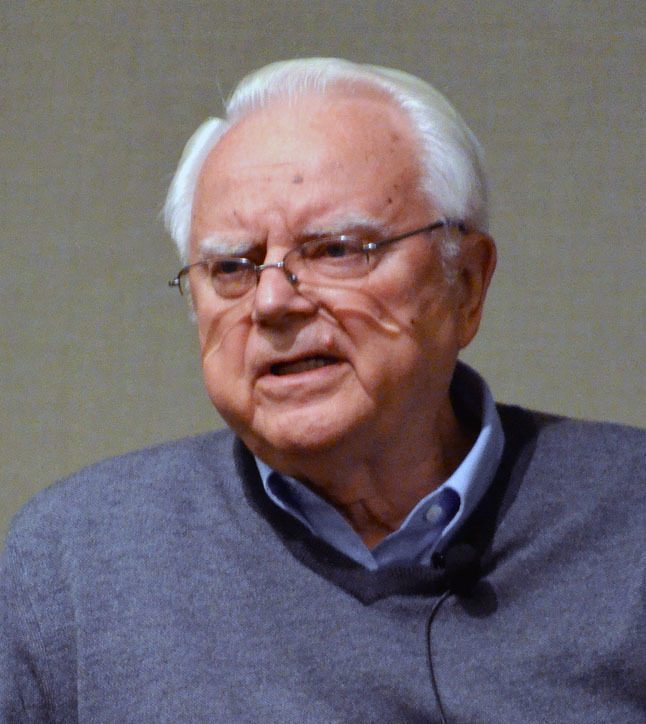
While he failed to find any evidence of a communicative civilization around Tau Ceti or Epsilon Eridani, Drake went on to a long and illustrious career in SETI. In 1974, he sent the Arecibo message to the globular cluster M13, about 25,000 light-years away. The message was a simple binary sequence, summarizing key information about humanity for the benefit of any aliens living there. Drake also collaborated with Carl Sagan to design the Pioneer plaques and the Voyager Golden Record, which, like the Arecibo message, were intended to communicate with alien intelligence, though these were actually physical objects sent out of the Solar System aboard NASA space probes.
The Drake equation
However, Frank Drake’s most famous contribution was the equation that bears his name. He devised it in 1961, right after Project Ozma, while he was preparing for a meeting of like-minded astronomers at the Green Bank Observatory; his focus wasn’t on extraterrestrial life in the abstract, but specifically on detectable civilizations that could be picked up with radio telescopes. The Drake equation is laid out as follows:
N = R(*) x f(p) x n(e) x f(l) x f(i) x f(c) x L
It looks simple enough, at first—just straightforward multiplication, from left to right. Here’s a breakdown of what the terms mean, as well as some possible values for them:
- N is the output of the Drake equation: the number of civilizations in the Milky Way galaxy with which we could potentially communicate.
- R(*) is the rate of star formation. In 1961, this was assumed to be about one per year, for the entire galaxy, though we now understand it as closer to four.
- f(p) is the fraction of stars with planetary systems. Drake placed this between 0.2 to 0.5; current estimates indicate that almost all stars have at least one planet orbiting them.
- n(e) is the average number of potentially life-bearing planets per planetary system. Drake’s guess was between one and five.
- f(l) is the fraction of those planets that develop life. Initially, this was assumed to be 100 percent.
- f(i) is the fraction of planets where primitive life forms evolve into mature technological civilizations. This was also 100 percent, in the original calculation.
- f(c) is the fraction of civilizations that yield detectable signs, such as radio signals. Drake assumed a low figure for this one: 10 to 20 percent.
- L is the length of time, in years, that an intelligent civilization can be expected to last. We have little idea what the average lifetime of other civilizations may be, but in the view of Frank Drake and colleagues, it was somewhere between one thousand and 100 million years. For comparison, the Roman Empire lasted around 1,500 years.
- N and n(e) are dimensionless numbers, while R(*) and L are in years^-1 and years, respectively, which cancel out. The rest of the components are expressed as percentages or fractions. They narrow down an initially large population of stars, like a series of sieves filtering debris from water, and each new step is only a tiny fraction as common as the previous step. Countless millions of potential stars are winnowed down to a relative handful of candidates.
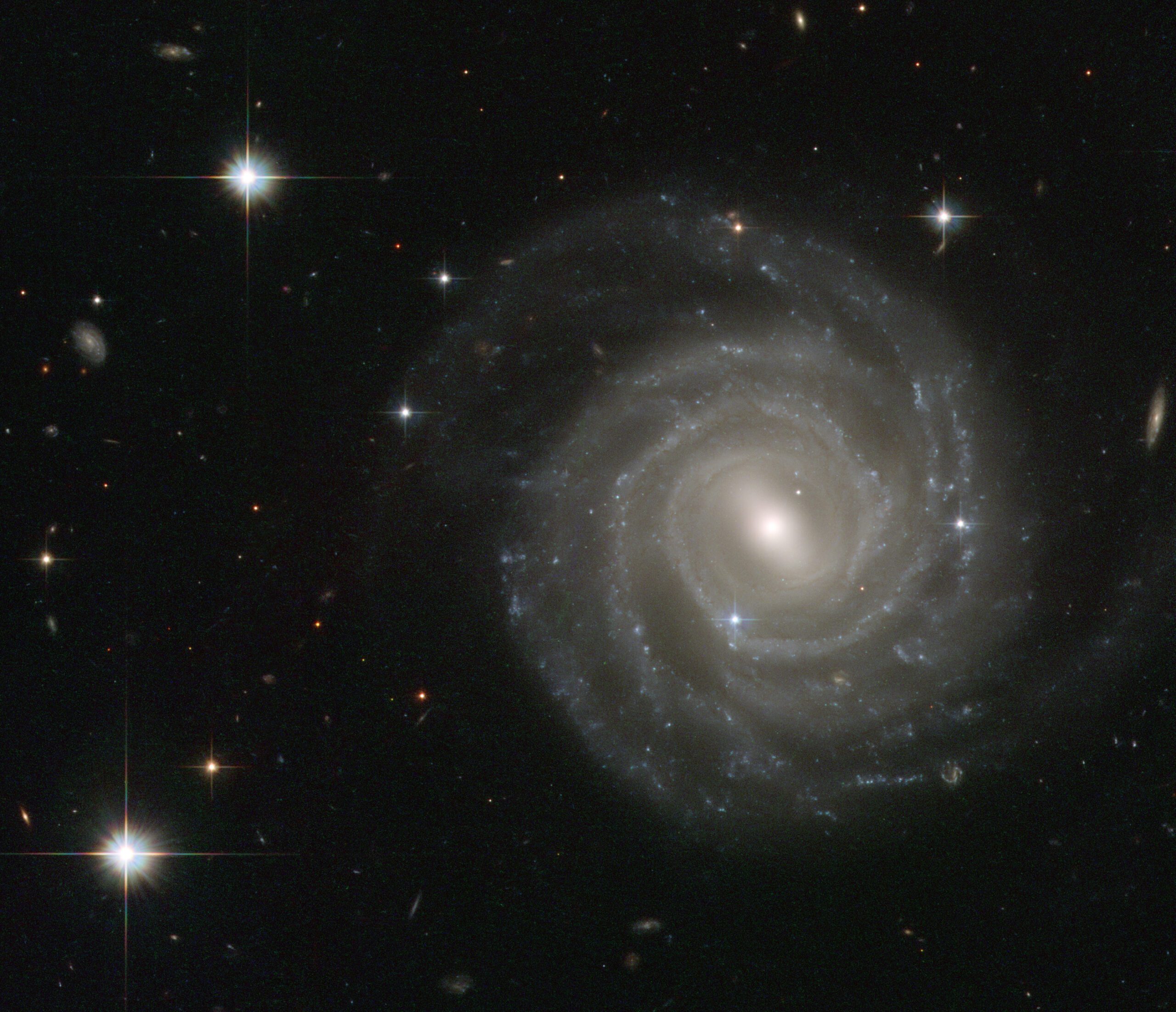
Educated guesses, and unanswered questions
The main problem with the Drake equation is that the inputs are poorly understood. We might be fairly sure that, say, the number of aliens depends on the probability of complex life on a given habitable planet. However, figuring out that probability is another matter entirely. And since the terms are multiplied together, any uncertainties compound. The final spread of possibilities is greater than that contained within any individual factor.
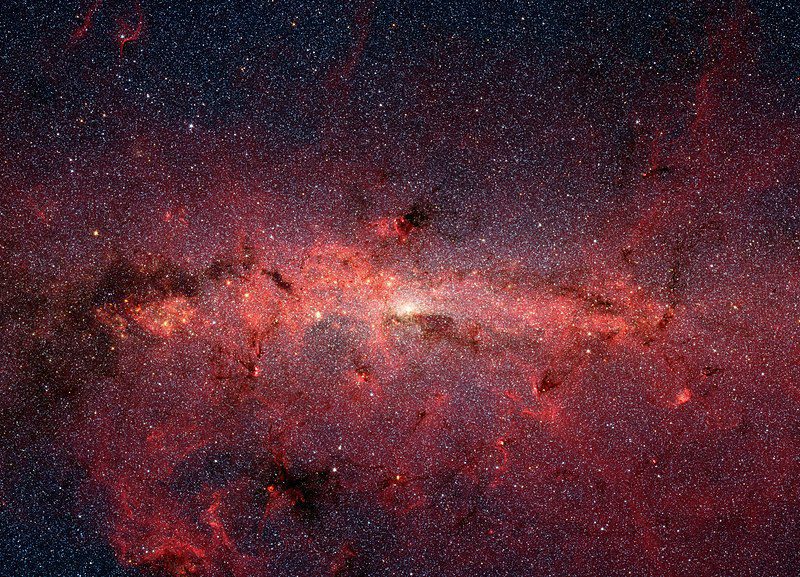
A formula like the Drake equation yields a very wide range for N. When Frank Drake and his colleagues did their initial calculation, using the values listed above, they produced a lower limit of 20 intelligent civilizations in the galaxy, and an upper limit of fifty million—not the most helpful result! They would have needed many more data points even to begin pinning things down.
The Drake equation has been picked apart many times over the years, and its underlying assumptions have come under scrutiny. Perhaps radio transmission is only one phase on the road to more sophisticated technologies, and everyone out there is talking via some method we can’t pick up. Perhaps some spacefaring civilizations are so dispersed that they are essentially immortal, making L more or less infinite. Alternately, f(i)—the fraction of life that goes on to develop intelligence—may be the key bottleneck; after all, life on Earth has existed for billions of years, but it has yielded only one sentient species.
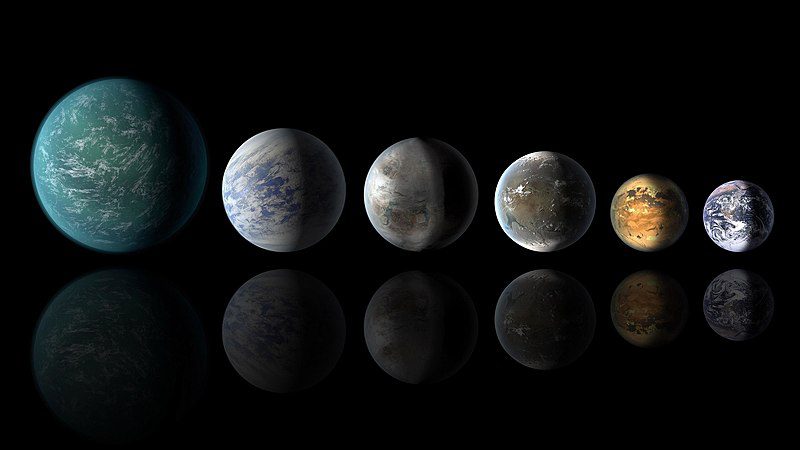
One of the most consequential unknowns is n(e), the prevalence of Earth-like planets per solar system. Despite recent advances in exoplanet hunting, which have shed light on thousands of worlds orbiting other stars, the fact remains that we don’t know quite how much of a rarity our homeworld really is. We also don’t know if conditions like ours are necessary to support life. The conventional understanding is that we should look for rocky planets like our own, orbiting around sun-like stars, in the so-called “habitable zone” where liquid water can exist on the surface—but what if life can arise in far stranger places? Perhaps many species evolve beneath the surface of cold, icy worlds, or use exotic chemicals like ammonia instead of water for their metabolism. We have hardly even begun to imagine the scope of what’s possible; when we try to calculate how often intelligent life emerges in the universe, we’re playing a game without knowing all of the rules.
Who’s out there?
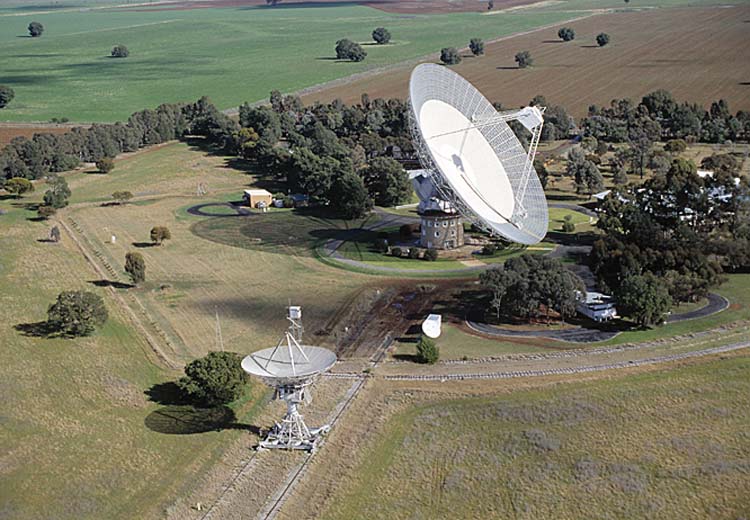
In the decades since it was invented, the Drake equation has profoundly influenced SETI efforts, speculative astronomy, and even science fiction. People have debated it relentlessly, proposing different values for its terms, and adding new ones altogether. Star Trek creator Gene Roddenberry used it as the basis for his famous TV series; when he first pitched the show in 1964, he cited it to support his premise of a galaxy teeming with intelligent life. Frank Drake himself was inspired by the knowledge that, statistically, there should be many extraterrestrial civilizations out there, and as noted above, he went on to spearhead many of the major SETI initiatives of the 20th century, including the Arecibo message and the Voyager Golden Record.
While we may not yet have any clear estimates for the universe’s population, Drake’s equation at least gives us a framework for understanding the problem. With some exceptions, we know what we need to know. As our understanding increases, with more surveys of extrasolar planets, and more extensive research into the mechanisms by which life evolves, a day may come when we can finally answer the vital question: are we alone?